The post-treatment separation in polymer production involves the removal of unreacted monomers or solvents, referred to as devolatilization. Devolatilization is a crucial step in the polymer production process, consuming 60% to 70% of the total energy. The results of this process directly affect the quality and performance of the final product.
Different types of devolatilization equipment can be used for polymer systems with varying viscosities. Typical equipment for high-viscosity fluid devolatilization can be categorized into the following five types based on their structural design: flash evaporation units, thin film evaporators (including falling film and agitated film types), falling liquid column or droplet evaporators (falling strip devolatilizers), surface renewal evaporators, and extrusion-type evaporators (vented extruders). From an economic perspective, twin-screw extruders have the highest energy consumption, while agitated film evaporators have the highest equipment cost. falling strip devolatilizers (FSD) have the lowest energy consumption and equipment cost. Combined, the total cost is about 40% lower compared to vented twin-screw devolatilizers or agitated film evaporators, making them the most widely used. Currently, most large-scale resin production plants using bulk polymerization processes employ FSD post-treatment technology. This article focuses on the latest research developments of FSD in recent years.
1. Characteristics of the FSD Devolatilization Process
In bulk polymerization processes, the monomer conversion rate is generally 50% to 80%, with 20% to 50% of the monomer needing to be removed in the devolatilization step. FSD differs significantly from vented twin-screw extruder devolatilization and conventional flash evaporation. The polymer-monomer mixture coming out of the polymerization reactor in the devolatilizer is a high-viscosity, multiphase system. During the devolatilization process, heat transfer, mass transfer, and flow are coupled together, with physical property data continuously changing, presenting nonlinear behavior. Different devolatilization mechanisms exist within the devolatilizer, making the understanding of FSD mechanisms, model establishment, and mathematical processing very challenging.
As devolatilization proceeds, the volatile content continuously decreases, the system viscosity continuously increases, and mass transfer becomes a challenge. High-temperature operations can easily cause polymer degradation or carbonization, affecting product quality. Discontinuous flow increases the difficulty of stable operation. These characteristics of the high-viscosity polymer devolatilization process make mathematical analysis of the devolatilizer more challenging than that of the polymerization reactor. Currently, understanding of this process is still largely empirical.
2. Characterization of the FSD Devolatilization Process
Different methods are used to characterize the devolatilization effects in constant temperature, uniform flow fields, and in variable temperature, non-uniform flow fields. Devolatilization fraction is a commonly used industrial characterization method. It can be analyzed using gas chromatography, ultraviolet spectrophotometry, polarography, and high-resolution liquid chromatography to determine the residual monomers and solvents in the polymer.
3. Devolatilization Structural Units
The technical features of FSD developed by various foreign companies are as follows:
(1) Monsanto Company: Uses a two-stage strip devolatilization, with a liquid level gauge controlling the liquid level of the first flash chamber to ensure the pressure difference between the two flash chambers. Suitable preheater and devolatilization conditions are chosen to ensure the mass fraction of low molecular weight products after devolatilization is <2% to 15%. The second-stage devolatilizer has two chambers with a recirculation line between them to enhance the devolatilization effect. Multi-layer sieve trays, contactors, and separation units receive the first-stage devolatilization vapor, while the second stage uses a liquid contact condenser.
(2) Dow Company: Utilizes a two-stage devolatilization, with the second stage being a rotating chamber with sufficient angular velocity to create a strong gravity field, separating the gas and liquid phases. Another type is a falling strip devolatilizer with a low shear mixer at the bottom of the flash chamber, particularly suitable for high-viscosity polymer systems, preventing severe product degradation. There is also a polymer devolatilization device including a flat plate heater and a gas-liquid collection and separation method. The flat plate heater consists of multi-layer grooved metal discs, with grooves of equal height but different widths, divided into conveyance, throttling, and divergent zones. The company also uses a recyclable stripping agent or introduces methanol to enhance the devolatilization effect.
(3) GE Company: Uses a preheater made of horizontally stacked metal discs, with the residence time of the polymer flowing through the preheater being 5 to 120 seconds, suitable for high-viscosity systems.
(4) Phillips Company: During devolatilization, 2% to 10% of a styrene-conjugated diene block copolymer by mass is added to the melt to improve devolatilization efficiency.
(5) Polysar Company: Installs a horizontal melt distributor in the falling strip devolatilizer to improve devolatilization efficiency. This distributor consists of an overflow weir and distribution disc, both with heating elements. The devolatilization efficiency is related to the foaming number of the polymer melt and the retention time of bubbles and strips in the devolatilizer. The melt first passes through a preheater at the top of the flash chamber maintaining an appropriately low pressure, and then through a horizontally placed distribution disc for devolatilization.
(6) Dainippon Company: Utilizes a tubular heat exchanger with a static mixer, with a distributor at the outlet of the heat exchanger, and adds a bubble nucleating agent to reduce the mass fraction of monomers in the polymer with a conversion rate of 80% to 95% to below 1%.
(7) Montedipe Company: The honeycomb heat exchanger inside the flash chamber has a heat exchange capacity of >80m²/(m³·hour), with the polymer flowing through the heat exchanger at a speed of <0.5mm/s and a residence time of 120 to 200 seconds, with the final volatile content mass fraction <5×10^-4.
(8) Union Carbide Company: Two-stage devolatilization, with a foaming agent added before entering the second stage to further improve devolatilization efficiency.
(9) Polymer Research Company and Cosdon Technology Company: Reintroduces volatiles after multi-stage devolatilization into the first-stage devolatilization zone and studies energy-saving and pipeline blockage prevention issues in the vacuum system.
(10) BASF Company: Uses one or more aids to remove vinyl aromatic hydrocarbons and/or vinyl unsaturated monomers. Minimizes the temperature difference on the heat transfer surface of the devolatilized melt and prevents the melt from cooling down during flashing, consisting of a set of metal plates with rectangular cross-sections, with the heat medium divided into several different temperatures and the width of the grooves adjusted to improve pressure drop in the groove.
(11) Novacor Company: Enhances devolatilization by selecting C₁4 ketones or alcohols that do not solidify at 0.672.67 kPa, with the final monomer mass fraction being less than 1.5×10^-4. The polymer solution passes through more than two shallow plate distributors with inclined pores, and a small amount of C₁~4 and CO₂ is added, resulting in a final monomer mass fraction of less than 1.5×10^-4. By adding more than 10% of the residual volatiles of water, the final monomer mass fraction is reduced to less than 5×10^-4, and steam is added between the devolatilizer and the vacuum source to solve the water solidification problem. Injects a non-oxidizing supercritical fluid with a mass fraction greater than 10% of the residual volatiles, achieving a final monomer mass fraction of less than 5×10^-4.
(12) Fina Company: Two-stage devolatilization, with the second-stage flash chamber forming strips through a series of nozzles.
(13) Badische Anilin & Soda Fabrik Company: Uses a preheater with a temperature gradient for the devolatilization of melts containing different volatile residual monomers and low boiling point products that are temperature-sensitive (e.g., acrylonitrile and styrene).
(14) Toyo Koatsu Company: Uses a conical device inside the chamber to disperse the melt.
FSD mainly consists of heat exchangers (sometimes acting as evaporators) and flash chambers. The design and operation of the heat exchanger are key to FSD. Research on strip devolatilization equipment mainly focuses on preheaters and fluid distributors.
(15) DODGEN Company: The principle of DODGEN DSXL devolatilization technology is to quickly and uniformly heat the polymer to a certain temperature through an efficient high-viscosity fluid heat exchanger, and/or add aids to the polymer to help volatiles escape, and then uniformly disperse the polymer in the devolatilizer, increasing the surface area of the polymer in the reactor, reducing the interfacial mass transfer resistance of volatile substances, achieving efficient devolatilization. Based on the characteristics of the polymer and the rich application experience in polymer devolatilization, DODGEN designs single-stage or multi-stage devolatilization processes, with core equipment including heat exchangers with mixing elements, mixers, and efficient distributors, achieving the final goal of improving product quality and reducing operating costs.
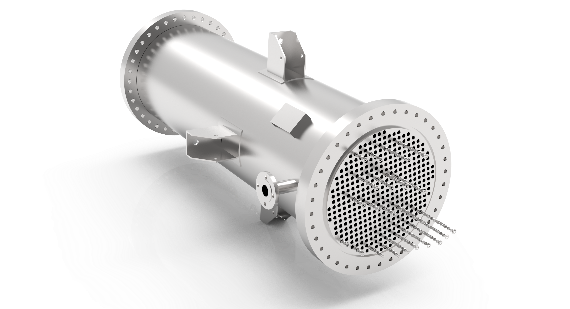
3.1 Preheaters
The design and operation of preheaters are crucial for enhancing the devolatilization efficiency of FSD. The primary distinction between different FSDs lies in the different structures of the preheaters used. A typical preheater is a single-channel tubular heat exchanger, with the shell side carrying high-temperature media such as thermal oil or steam. In recent years, patents related to FSD have placed significant emphasis on preheaters. Preheaters with static mixer elements in the tubes, those with a shell-side temperature gradient, preheaters with narrow radial melt slots, and those composed of horizontally stacked disc melt slots, as well as honeycomb preheaters placed inside the flash chamber, all use different methods to uniformly heat polymers in a short time, playing a positive role in preventing polymer degradation.
The operational efficiency of FSD depends to some extent on the uniform distribution of the melt in the preheater. Typically, a distributor is installed at the top of the preheater, allowing the melt to enter the chamber through a spinneret, slit distributor, or overflow weir, thereby increasing the specific surface area. Additionally, the elongation or rupture of the melt as it falls can result in surface renewal, significantly improving the devolatilization efficiency.
3.2 Flash Chamber
Flash chambers usually have a jacket to provide heat using thermal oil or steam, or they use electric heating to ensure the necessary surface temperature during startup and to supplement heat lost to the environment.
Maintaining a certain liquid level inside the FSD chamber is also essential. It ensures the required vacuum level inside the chamber and allows the discharge pump to function correctly. It also significantly impacts product quality. Excessive residence time can lead to polymer degradation and carbonization. However, for rubber-toughened products, the residence time at high temperatures post-devolatilization can promote a moderate level of thermal cross-linking between rubber molecular chains, stabilizing the morphology of rubber particles and ensuring good impact resistance after shearing.
The liquid level of the melt inside the FSD chamber is typically controlled by an automatic system, operated by adjusting the discharge pump speed. A sight glass can also help control the liquid level. Coil pipes can be installed in the liquid accumulation area to provide additional heat transfer, reducing the temperature drop caused by the evaporation of volatiles. However, this is only suitable for non-crosslinking or non-thermally degrading polymer systems.
3.3 Melt Discharge Pump
Both gear pumps and screw pumps can be used, but they must have large feed openings and be able to operate under vacuum conditions.
3.4 Condensation and Vacuum System
Lowering the temperature of the superheated steam can enhance condensation capacity. This can be achieved by passing the steam through a superheated steam cooler connected to a circulating refrigeration jet port, followed by passing the saturated steam through a single-shell tubular condenser to achieve subcooling. In industrial production, multi-stage steam jet pumps are commonly used for the vacuum system, while mechanical vacuum pumps are less frequently employed.
4 Measures to Enhance Devolatilization
4.1 Enhanced Operating Conditions
In industrial applications, the degree of superheat is commonly defined as H₈ = Pi - Po, where Pi is the saturation vapor pressure of the volatiles in the devolatilization system, and Po is the total pressure within the vacuum chamber. In a given system, Pi is largely a function of temperature and volatile concentration. In practical operations, maintaining as high a degree of superheat as possible can improve devolatilization efficiency. Several measures can achieve this goal:
Increasing the melt temperature to raise Pi. However, this is limited by heating capacity and must consider polymer crosslinking, degradation, cracking, and oligomer formation.
Reducing the temperature drop caused by evaporation. The design of the preheater and additional heat exchange surfaces within the FSD chamber can limit the drop in Pi due to the evaporation of volatiles.
Reducing the FSD chamber pressure Po, though this is constrained by the cost of vacuum equipment.
4.2 Addition of Low-Boiling Devolatilization Aids
Adding substances such as water (steam), methanol, CO₂, N₂, benzene, or other low-boiling substances can reduce the partial pressure of the volatiles, thereby lowering the equilibrium concentration of volatiles in the melt and increasing the devolatilization concentration gradient. The key to this technique is the selection and design of online mixers; otherwise, the effect may be suboptimal.
4.3 Ultrasonic and Other Enhanced Devolatilization Methods
When the volatile content in polymers is very low, bubble nucleation becomes a bottleneck in the devolatilization process. Strong ultrasonic waves can induce high-frequency tensile and compressive stresses in the liquid, disrupting its continuity and creating small bubble regions (acoustic cavitation), thereby enhancing devolatilization. Supercritical extraction and microwaves are also new technologies being applied to devolatilization research. Supercritical extraction overcomes the degradation issues associated with traditional latent heat-dependent polymer devolatilization methods and is highly effective for separating high-boiling, low-volatility, and heat-sensitive substances.
4.4 Multistage Devolatilization
Single-stage devolatilization usually cannot meet devolatilization requirements, especially during the foaming stage of FSD. The cooling effect brought about by large-scale evaporation slows down the devolatilization rate. Additionally, the large amount of steam generated requires a large-volume flash chamber and steam pipelines to match, increasing the load on the vacuum system. Therefore, when the devolatilization ratio exceeds one to two orders of magnitude, multistage devolatilization should be considered. Generally, two stages are suitable for balancing investment and devolatilization effect. With two-stage devolatilization, the reduction in condensation capacity demand and steam pipeline investment often exceeds the investment in two flash chambers. Each stage can be optimally designed and operated, providing greater devolatilization capacity and operational flexibility, suitable for different volatile content and monomer residue requirements. It also effectively reduces the exposure time of the devolatilized melt at high temperatures, lowering the possibility of polymer decomposition.
4.5 Surface Renewal
For high-viscosity devolatilization systems, surface renewal of the material in the flash chamber can improve the diffusion coefficient and enhance devolatilization.
5 Conclusion
FSD equipment is simple, with low investment, convenient operation, and easy diagnosis and correction of issues, making it particularly suitable for devolatilizing polymers where foaming devolatilization control is required. Therefore, it has been increasingly used in industrial installations, especially in bulk polymerization plants. In the domestic market, several imported styrene series bulk polymerization plants have adopted FSD for post-treatment. The technologies imported by Takahashi Chemical Plant, Lanzhou Petrochemical Company, and Jilin Chemical Group from TEC-MTC in the 1980s, and the technology imported by Yanshan Petrochemical Company from DOW, use single-stage FSD. The technology imported by Qilu Petrochemical Company from TEC-MTC in the 1990s, and the technology imported by Fushun Petrochemical Company from COSDON, use two-stage FSD. However, FSD also has drawbacks, such as relatively long average residence time and poor fluidity, which limit its application to some extent.
Improving the structure of falling strand devolatilizers, developing new types of FSD devolatilization equipment and methods with compact structure, fast surface renewal, and high heat and mass transfer efficiency, such as designing preheaters that accelerate in-situ evaporation to minimize condensation caused by evaporation. This includes researching the shape and structure of melt slots in preheaters and innovating the distribution and circulation of heating media. Further development of online mixing equipment suitable for volatile additives; coupling a mechanical power devolatilizer such as a thin-film evaporator or a vented twin-screw extruder as a second-stage devolatilizer; the application of supercritical fluid and microwave ultrasound technology; and simulating and optimizing existing devolatilization processes to obtain optimal operating parameters, reduce energy consumption, and improve economic benefits, will enable FSD to demonstrate its superior functionality and have an increasingly promising application prospect.
[References]
[1]王凯,孙建中.工业聚合反应装置[M].北京:中国石化出版社,1997,271~280.
[2]潘勤敏,刘青.[J].合成橡胶工业,1998,21(4):198~202.
[3]谢建军,潘勤敏,潘祖仁.[J].合成橡胶工业,1998,21(3):135~141.
[4]Ramon JA.Polymer Devolatilization [C].New York:Academ-ic Press,1996.
[5]Gordon R E,McNeill G A.Falling Strand Devolatilizer Using One Preheater with Two Flash Chambers [P].US:3853672, 1974—12—10.
[6]Hagberg C G.Falling Strand Devolatilization Technique [P].US:3928399,1975-12-23.
[7]Newman R E.Falling Strand Devolatilizer [P].US:4294652,1981—10—13.
[8]Bir WG,Novack J.Recovery of Alkenyl-aromatic Monomers by Falling Strand Devolatilization [P].US:3884766,1975-05 —20.
[9]Bir WG,Novack J.Recovery of Alkenyl-aromatic Monomers by Falling Strand Devolatilization [P].US:3886049,1975—05 —27.
[10]Eugene R.M,Robert A H.Method for the Devolatilization of Thermoplastic Materials [P].US:4952672,1988—08-11.
[11]Eugen 万 方 数 据el T E.Apparatus and Process for De- volatilization of High Viscosity Polymers[P].US:4954303,1990—09—04.
[12]Cummings C K,Meister B J.Polymer Devolatilizer [P].US:5453158,1995—09—26.
[13]Weller JP,Wilson L D.Polymer Devolatilization [P].US:5861474,1999—01—19.
[14]Fujimoto S.Devolatilization of Alkenyl Aromatic Polymers[P].US:3987235,1976-10-19.
[15]Aneja VP,Skibeck JP.Method for Devolatilizing Polymer Solutions [P].US:4808262,1989—02-28.
[16]Farrar J Ralph C,Hartsock D L,et al.Reduction of Residual Volatiles in Styrene Polymers [P].US 5185400,1990—0209.
[17]Aboul N,OsmanT.Distributor for a Devolatilizer [P].US:4934433,1991—12—03.
[18]Aboul N,Osman T.Devolatilization [P].US:4934433,1990—06—19.
[19]Morita T,Shimazu K,FuruKawa M.Devolatilization of Liq- uid Composition Containing Polymer and Volatile Con- stituents [P].US:5024728,1991—06—18.
[20]Mattiussi A,Buonerba C,balestriF,et al.Process for the De- volatilization for Polymer Solutions [P].US:5084134,1992— 01—28.
[21]Nauman E B,Szabo T T,Klosek FP,et al.Devolatilization of Liquid PolymerCompositions [P].US 3668161,1972—0606.
[22]McCurdy JL,Jarvis M A.Apparatus for the Multiple Stage Devolatilization of Mass Processable Polymers [P].US:4383972,1983—05—17.
[23]McCurdy JL,Jarvis M A.Multiple Stage Devolatilization Process for Mass Processable Polymer [P].US:4439601,1984—03—27.
[24]Reffert R W,Hambrecht J,Jung R H,et al.Treatment of Copolymers to Remove Residual Monomers [P].US:1985— 03—05.
[25]Fink P,Wild H,Zizlsperger J,et al.Process and Apparatus for Removing Vaporizable Constituents from Viscous Solu- tions or Melts of Thermoplastics [P].US:4153501,1979-05 —08.
[26] Skibeck J P.Fluid Assisted Devolatilization [P].US:5350813,1994—09—27.
[27]Krupinski S M,Desroches D.Devolatilizer Tray Array [P].US:5874525,1999—02-23.
[28] Skibeck J P.Water Assisted Devolatilization [P].US:5380822,1995—01—10.
[29]Krupinski S M.Devolatilization [P].US:5691445,1997-11—25.
[30]Sosa JM,Scates RM,Weguespack JN,et al.Method for Re- ducing Volatiles in Polymerized Styrene [P].US:5540813,1996—07—30.
[31]Metzinger L,Gotschalk A.Process for the Removal of Volatiles from Polymer Solutions [P].US:3865672,1975— 09—26.
[32]Kimoto K,Yamagisawa Y.Process and Apparatus for Re-moving Volatile Substances from Viscous Compositions [P].US:3694535,1972—09-26.
[33] 岳传龙,陈光银,朱文炫.[J]. 塑料工业,1990(5):18-19.
[34] 魏丹毅,蒋春跃.[J]. 化学工程,1999,27(3):5-7.
[35] 蒋春跃,潘勤敏,潘祖仁.[J ].合成橡胶工业,1996,19(5):303.