Under the "dual carbon" goals, a series of implementation guidelines have been introduced, unveiling vast potential in emerging blue oceans. Achieving carbon neutrality can be approached from three key directions: energy generation, energy consumption, and resource recycling. Examples include replacing non-renewable petroleum with clean energy, adopting production processes and equipment that emit less CO₂, and recycling and reusing conventional plastic products. Among these various pathways, synthetic biology stands out as particularly promising.
The New Blue Ocean of Synthetic Biology
Global carbon emissions primarily stem from energy consumption and material usage. Approximately 500 million tons of polymer materials are consumed annually, with each ton releasing 8–9 tons of CO₂. This means humanity generates a staggering 4–5 billion tons of CO₂ solely from polymer materials, posing significant challenges to environmental sustainability and global development.Synthetic biology offers a low-carbon advantage on both the energy generation and consumption fronts. By leveraging synthetic biology to construct microbial cell factories, renewable biological resources such as sugars can be transformed into a wide range of bulk chemicals. This enables the green and clean production of bulk chemicals, reducing dependence on petroleum resources and addressing the high energy consumption and pollution associated with petrochemical manufacturing.Furthermore, using biochemical or biological methods to produce chemicals has emerged as a new manufacturing niche, reshaping business strategies and influencing consumer perceptions.
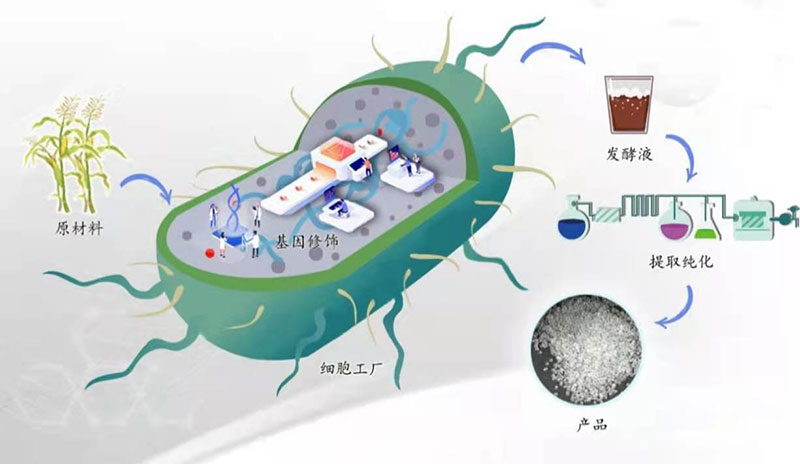
A group of synthetic biology companies (e.g., Ginkgo, Amyris, Zymergen, Beam, etc.) is experiencing rapid growth. According to data from Transparency Market Research, the global synthetic biology market reached $4.96 billion in 2018 and is projected to exceed $40 billion (260 billion RMB) by 2027.
Cell factories constructed using technologies such as genome editing, multi-gene regulation, protein scaffolding, and high-throughput screening have enabled the production of a variety of bulk chemicals. The influx of capital has empowered more synthetic biology service companies to scale up production and bring to market products with significant potential.
Synthetic biology has broad application prospects in fields such as bio-based chemicals, biomaterials, and bioenergy. Among them, three categories of bulk chemicals—organic amines (e.g., cadaverine, putrescine, caprolactam), organic acids (e.g., succinic acid, lactic acid, malonic acid, L-malic acid, adipic acid), and organic alcohols (e.g., 1,3-propanediol, 1,4-butanediol, isobutanol)—have particularly wide-ranging applications.
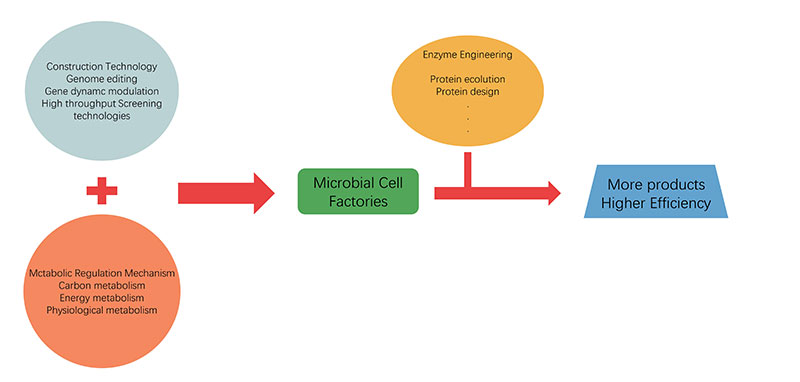
With the advancement of technology, breakthroughs have been made in the construction of cell factories and the design of metabolic pathways for fermentation processes. However, the separation and purification of biosynthetic products remain a bottleneck for the industrialization of synthetic biology. Industry experts point out that the challenge of using “biological methods to replace chemical methods” for producing bulk chemicals lies not in conversion but in “purification.” After fermentation, there are numerous impurities inside the cells. For high-molecular polymerization, the raw materials must be exceptionally pure. However, biological cells are extremely complex and diverse. While they can produce specialized chemical materials with superior performance that chemical methods cannot synthesize, they also generate a variety of metabolites, leading to low yields of the target product. After all, it is challenging to fully understand the intricate details of all the transformations during fermentation. Enhancing the purity of the target product further increases costs, making the cost of biomanufacturing significantly higher than that of chemically producing similar products.
How to Choose the Right Separation and Purification Method
For synthetic biological chemical manufacturers, only by producing high-purity products can they be widely adopted by downstream users and achieve profitability through economies of scale. For manufacturers, when producing high-value-added products on a smaller scale, the priority is to quickly bring the product to market, with separation methods that meet purity requirements being the first choice. Once the product can be mass-produced, cost-reducing separation methods should take precedence. For bulk chemicals produced on a scale of tens of thousands of tons, a combination of multiple unit operations is typically employed for separation. The diagram below illustrates the technological maturity of different separation techniques, such as distillation, absorption, crystallization, membrane separation, and chromatography.
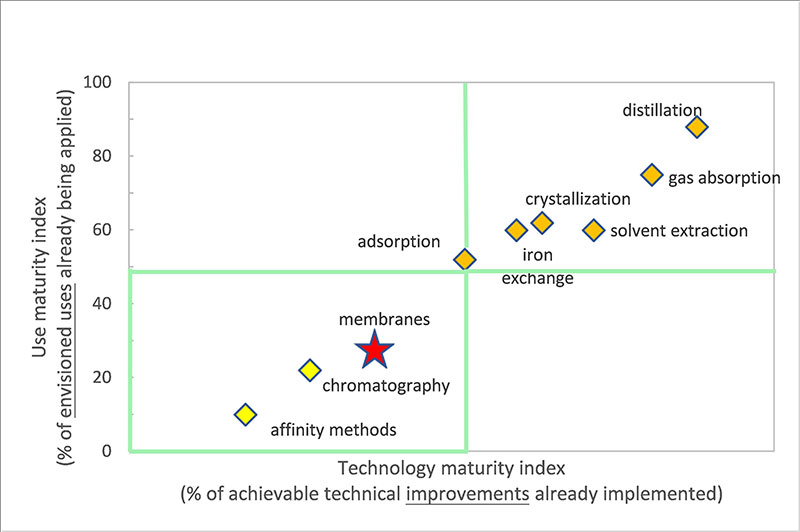
For companies producing chemicals using synthetic biology methods, relying on traditional separation and purification equipment makes it challenging to meet high-purity separation requirements. Additionally, heat-sensitive substances tend to decompose at high temperatures, and the energy consumption and operational costs associated with high-temperature operations are significant concerns for these companies. For instance, the traditional method of separating cadaverine involves extraction-distillation, achieving a purity of 99.19%[1]. In contrast, the new separation method—melt crystallization—separates cadaverine through the processes of crystallization, sweating, and melting, achieving a product purity of 99.92%[2], effectively meeting polymer-grade requirements. Moreover, melt crystallization operates at low temperatures, which prevents chemical changes in heat-sensitive substances and significantly reduces energy consumption.
[1] Study on l-Lysine Synthesis to Cadaverine Catalyzed by Recombinant E. coli Bl-DAB and Its Purification [D]
[2] Cadaverine Melt Crystallization Process [P]
Another example of high fermentation efficiency but bottlenecks in separation and purification is the production of polylactic acid (PLA). The purity of lactic acid significantly impacts its cost, ultimately determining the price of PLA. Developing a new generation of lactic acid purification technologies is the key to overcoming this bottleneck. DODGEN has adopted a coupling of multiple separation techniques, aiming to reduce the cost of PLA to below $2,000.Our pilot modular plant in Ningxia has conducted industrial-scale validation of various coupled separation technologies, contributing to breakthroughs for multiple synthetic biology companies domestically and internationally.
Unlocking the Accelerator for Process and Equipment Innovation
Synthetic biology has revolutionized chemical production, and the rapid development of related enterprises domestically and abroad has boosted market confidence. Accelerating product industrialization is imperative, and advanced, experienced partners in the separation field serve as key accelerators in this process.
DODGEN acts as a catalyst for driving innovations in production processes and equipment. With comprehensive solutions in the separation field, we provide cost-effective and efficient solutions to help clients choose reliable separation methods. Our R&D center is future-oriented, leveraging robust scientific and technological capabilities to enhance separation efficiency through the coupling of multiple separation techniques, while also developing innovative process equipment tailored to the needs of synthetic biological chemicals.In the field of synthetic biology bulk chemicals, we possess extensive experience in downstream separation and purification stages. In addition to offering advanced separation solutions, we provide pilot-scale industrial validation services to help our clients accelerate product industrialization. This not only strengthens their competitive position in the market but also enables them to seize future development opportunities.
If you are interested in our green separation technology, please scan the QR code below for more information.
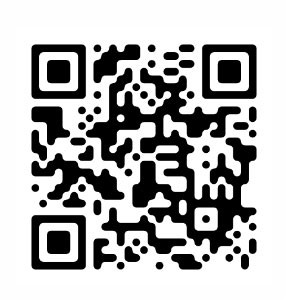
If you are interested in our melt crystallization technology, please scan the QR code below for more information.
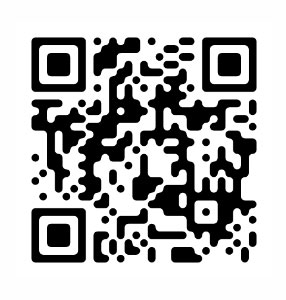